1.2. MOLECULES
Names, formulas, models
In the chemical formulas used to indicate molecules, the number of atoms of the same type that are part of a molecule is indicated by a subscript. For example, the oxygen molecule, formed by two oxygen atoms, is represented by the formula O2; the water molecule is made up of two hydrogen atoms and one oxygen atom, and is thus represented by the formula H2O. Many molecules are composed of a greater number of atoms. For example, the molecule of acetic acid (Fig. 2a, left) consists of 8 atoms, of which two carbon, four hydrogen and two oxygen: C2H4O2. There are, however, many more complex molecules, such as vitamin B12, which is composed of 181 atoms: C63H88CoN14O14P (Fig. 2b, left).
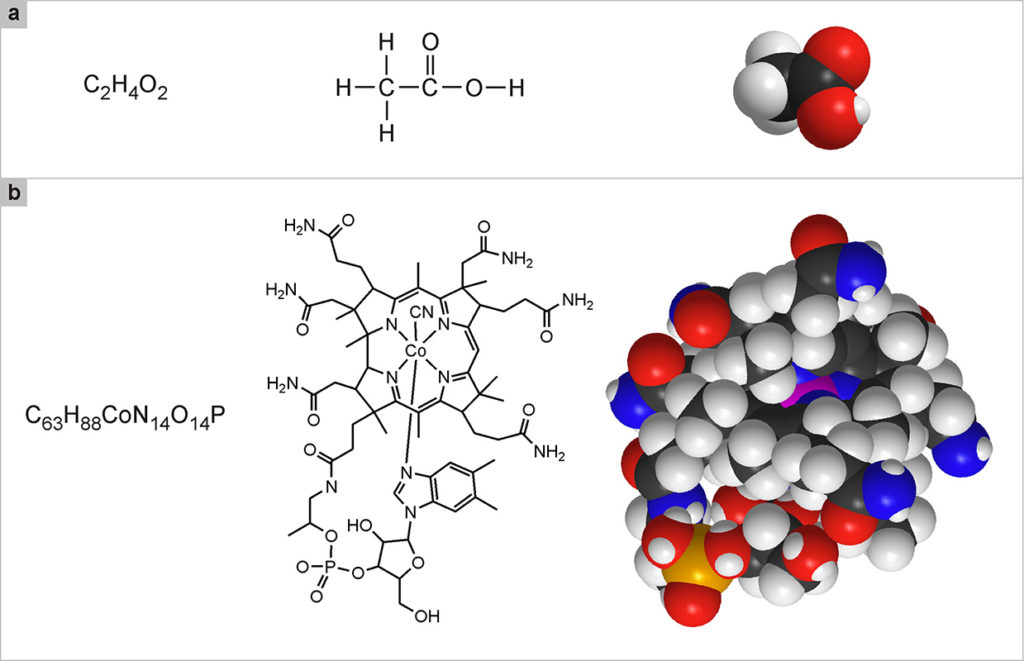
Like animals and plants, molecules have common names (water, acetic acid, vitamin B12) and scientific names (in the case of water, dihydrogen monoxide). The scientific names of large molecules are extremely complex and therefore are hardly ever used. Since the names are not enough to get oriented in the enormous and variegated world of molecules, it is necessary to resort to another type of representation; chemical formulas. H2O, C2H4O2 and C63H88CoN14O14P, are called brute formulas and indicate only which and how many atoms the molecule is composed of. These formulas are not very useful because they do not specify which atoms are bonded to which, nor do they specify their spatial arrangement. For example, 6 carbon atoms and 6 hydrogen atoms can be combined in 217 different ways, which means that the same formula, C6H6 corresponds to as many as 217 different molecules, of which the best known is benzene. This example also makes it clear that, with the hundreds of atomic species available, it is possible to obtain a huge number of molecules. Therefore, we often resort to structural formulas, that highlight how the various atoms are linked together.
In these formulas, the bonds are the “glue” that holds the atoms together, and are represented by dashes that unite the symbols of the connected atoms. For small molecules, the structural formulas are simple and, along with clearly indicating how the atoms are linked, they also give an idea of the shape of the molecule (Fig. 2a, center). For large molecules, the situation becomes increasingly complex and the structural formulas end up looking like an intricate web of signs. In these cases, we try to represent the molecule with simplified structural formulas (Fig. 2b, center): for example, carbon atoms, C, which are very common especially in the molecules of living organisms, are no longer explicitly indicated in the structural formula, but it is understood that they occupy the intersecting positions of the dashes that indicate the links. Likewise, the hydrogen atoms, H, bound to the carbon atoms, and the dash that represents their bond, are not indicated.
Although very useful to scientists, structural formulas are not known for their attractive qualities. The most realistic and significant way to represent the molecules is based on the use of three-dimensional models, enormously enlarged, but in scale, compared to reality. These models are built with an interlocking mechanism similar to the one used in the well-known Lego game, starting from rigid plastic spheres that represent the various types of atoms, with small cavities in which junctions representing the chemical bonds can be inserted. Each sphere representing an atom is one hundred mil-lion times larger than the real size of the corresponding atom, so that the model is to scale and therefore accurately represents the relative dimensions of the various molecules and the parts that constitute them. To distinguish the various types of atoms, or rather the most recurring ones in important molecules, conventional colors are used: white for hydrogen (H), black for carbon (C), red for oxygen (O), blue for nitrogen (N), yellow for sulfur (S), orange for phosphorus (P), green for chlorine (Cl).
Represented with three-dimensional models (Fig. 2, a and b, on the right), the molecules appear as macroscopic objects and acquire part of the charm they would have if we could see them in their reality. In the case of very large molecules, however, molecular models are also difficult to decipher. As we will see further on, an ultimate way to represent the most complex molecules and the aggregates of molecules that make up molecular devices and machines is to use schemes of various types that help clarify the shape, properties and functions of these systems.